Research projects
Research interests
We are interested in the correlation of structural, electronic, and magnetic properties of surfaces and nanostructured materials. Our main experimental methods are (spin-polarized) scanning tunneling microscopy (STM). By varying, for example, the microscope temperature or the applied external field these techniques are applied in various environments.
Our research program is based on the three columns below:
Atomic scale transport properties
The Molecular Nanoprobe (MONA)
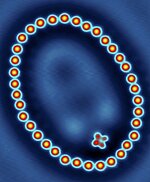
The electrical resistance of materials or devices is often measured by the 4-point probe method to eliminate lead and contact resistance issues. In the recent decade, miniaturized versions, so-called nanoprobes, have been developed where four sharp tips are individually positioned by piezo-actuators and simultaneously imaged with a scanning electron microscope (see here for an example). However, the finite tip sharpness usually limits the minimal distance to about 100 nm.
We recently developed of a novel method which enables to detect how charged quasiparticles propagate on length scales down to a few nm by remotely triggering the tautomerization of a single molecule with a scanning tunneling microscope (STM) (see here and the highlight in Nature Nanotechnology for further information). In analogy to the 4-point nanoprobe we coined it “molecular nanoprobe” (MONA). In combination with atom-by-atom-engineered interferometers, MONA allows to unravel the quantum-mechanical wave nature of hot electrons. Two interferometers can even be combined to build an energy-dependent selector, which allows it to selectively switch one out of two molecules without changing the position of the STM tip. The MONA technique may, in future, serve as a method to map the charge density distribution around an arbitrary quasiparticle injection point.
Imaging magnetic nanostructures
Spin-Polarized Scanning Tunneling Microscopy (SP-STM)
The understanding of magnetically ordered states in solid-state materials is an exciting field of research. Nowadays, advanced surface analysis and microscopy tools allow for the detection of magnetic signals with unprecedented sensitivity and spatial resolution. In this context, spin-polarized scanning tunneling microscopy (SP-STM) is of particular interest as it enables to image the sample's spin structure with atomic resolution. For example, SP-STM allowed for the first direct imaging of antiferromagnetic surfaces and domain walls and of frustrated Nèel spin states. Furthermore, it turned out that the spin-orbit–induced Dzyaloshinskii-Morija interaction (DMI) can be very significant at surfaces and interfaces. As a result, spin structures with increasing complexity were detected. Whereas collinear ferro- or antiferromagnetism governed dominated the scientific debate in the past, we have witnessed a plethora of more complex non-collinear magnetic states since the advent of the current century, such as non-collinear antiferromagnets and chiral spin cycloid.
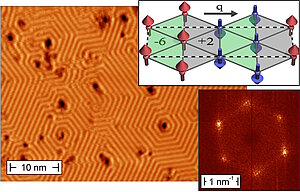
Topological materials
Unraveling twisted electronic states
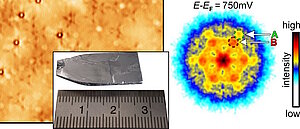
The discovery of topological insulators (TIs) about 10 years ago led to intense research efforts towards the utilization of these materials in spintronic, magneto-electric, or quantum computation devices. We employ several scanning probe-based techniques, such as scanning tunneling spectroscopy (STS), quasiparticle interference (QPI), or Landau level spectroscopy (LLS), to investigate the correlation between dissipation-less quantized transport and the onset of ferromagnetism in three-dimensional (3D) TIs. In the meantime, we studied a wide range of bulk- and surface-doped 3D TIs. Our results paint a consistent picture of how and under which conditions these dopands lead to long-range magnetic order which breaks time- reversal symmetry. For example, we find that the fate of the topological surface states critically depends on the TM element: while V- and Fe-doped Sb2Te3 display resonant impurity states in the vicinity of the Dirac point, Cr and Mn impurities leave the energy gap unaffected.